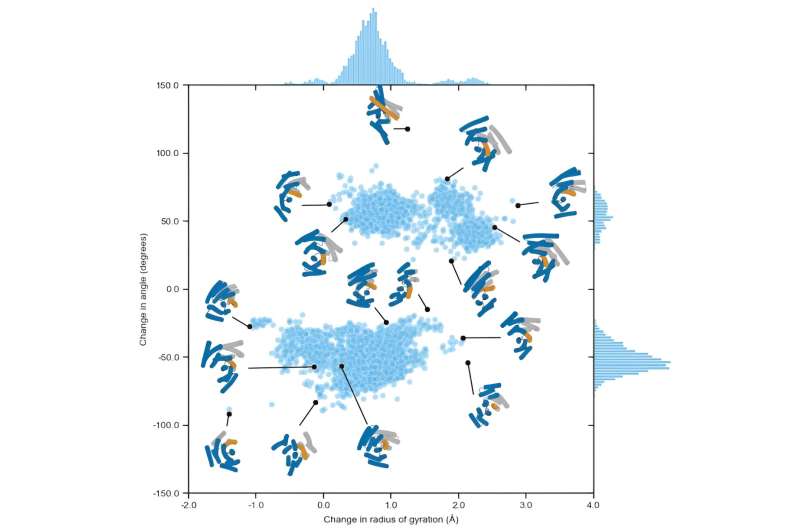
Scientists from the University of Washington have designed custom proteins that can switch between two fully structured conformations. By coupling this molecular motion with a binding event, these proteins serve as biological counterparts to electronic transistors, opening the door to new applications in smart therapeutics, environmental sensing, and more. The study has been reported in the journal Science
A team led by postdoctoral scholars Florian Praetorius, Ph.D., and Philip Leung, Ph.D., used a combination of deep learning and physics-based algorithms to design custom proteins with two distinct but fully structured conformations. In collaboration with the IPD Crystallography Core and the Stoll Lab in the Department of Chemistry, the team determined the structures of the proteins and measured how they move. Analyzing their kinetic and thermodynamic properties allowed them to fine-tune the molecular motion.
“Before, we could only create proteins that had one stable configuration,” says Praetorius. “Now, we can finally create proteins that move in a predictable way, which should open up an extraordinary range of applications.” The new proteins are already being incorporated into other research projects in the lab, showcasing their versatility as key building blocks for future biotechnologies.
Switching things up
Creating proteins that toggle between two shapes required a new approach to multi-state design. In the past, multi-state design has yielded proteins that make very small shape changes, peptides that switch shapes based on the presence of metal ions, and similar sequences that can fold into very different shapes.
The team’s stimulus-responsive LOCKR proteins were designed to “open” upon target binding, but while the “closed” state of these molecular switches is a well-defined shape, the “open” state can take on a wide variety of conformations. LOCKR technology has been used to create biosensors, but the undefined second state makes it less suitable for tasks that require mechanical coupling, such as in a molecular machine or computing systems that work based on discrete states.
Using a combination of Rosetta two-state design and ProteinMPNN, the team generated amino acid sequences that can fold into more than one well-defined structure. Designing one of the resulting conformations to bind to a target peptide or protein couples the conformational equilibrium with target binding, allowing the possibility of “toggling” the protein shape upon binding. This strategy should enable protein design to go beyond static structures to more complex multistate assemblies and machines.
Biological transistors
“We believe these hinge proteins are akin to the transistors in electronics,” explains Leung. “Just as transistors respond to and control the flow of electrical signals, these proteins can change shape to control biological interactions at the molecular level.”
For instance, in one state, the protein might be inactive or have one type of function, and in the other state, activated by the binding event, it might have a different function. This capability to switch between states allows these proteins to dynamically respond to their biological or chemical environment, akin to how transistors allow for dynamic control of electronic signals.
The development of transistors revolutionized electronics, paving the way for the digital era. The researchers believe that the design of proteins that can switch between different states in a controlled way can also revolutionize biotechnology, enabling new kinds of responsive biomaterials, biosensors and smart drug delivery systems.
More information:
Florian Praetorius et al, Design of stimulus-responsive two-state hinge proteins, Science (2023). DOI: 10.1126/science.adg7731
Provided by
University of Washington
Citation:
Researchers design switch-like proteins inspired by transistors (2023, August 23)
retrieved 26 August 2023
from https://phys.org/news/2023-08-switch-like-proteins-transistors.html
This document is subject to copyright. Apart from any fair dealing for the purpose of private study or research, no
part may be reproduced without the written permission. The content is provided for information purposes only.